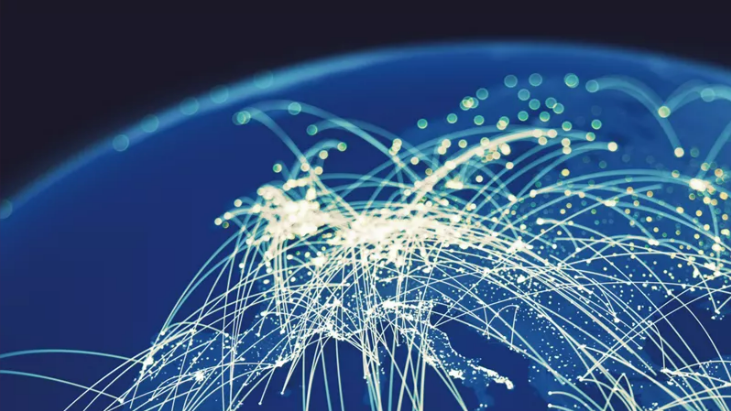
Sometime around 490 BC, the Greek runner, Pheidippides, is said to have sprinted from Marathon to Athens, about 26 miles, to announce the Athenians’ victory over the Persians. He then promptly expired, becoming one of the earliest and most famous casualties of the speed vs distance conundrum. To be fair, myocardial infarction with non-obstructive coronary artery disease may have been the cause of his death. So, we can add inadequate capacity of the heart and lungs to the equation as well.
While admittedly a loose analogy, Pheidippides’ failed attempt illustrates the challenge of optimizing distance, speed and capacity simultaneously—a problem all too familiar (and confounding) for today’s internet cloud providers (ICPs) and data center operators (DCOs).
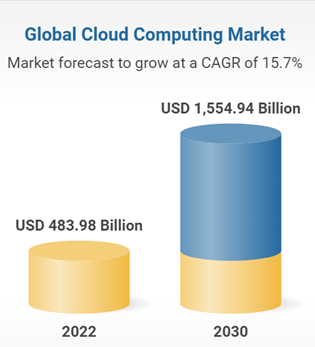
At the heart of the issue are the rapid changes in regional data center ecosystems combined with the on-going migration to the cloud. As ultra-reliable, low-latency communication (URLLC) applications move to the network edge, hyperscalers look to establish a more localized presence in key markets. This takes a variety of forms, from partnerships with co-lo providers to edge-based data center pods. Virtualized cloud architectures play a major role in enabling these changes, providing huge economies of scale and efficiency.
These shifts in resource allocations are having a profound impact on data center interconnects (DCIs) as fiber demand and overall capacity requirements continue to increase. ICPs and DCOs are under increasing pressure to help customers address the exploding needs for more data and cloud services in all parts of the network. This will include adopting new technologies in both line systems and optical transmission to enable ultra-high capacity, massive scalability, power efficiency, and lower cost per bit.
Before we look at what’s possible, let’s take stock of where we are from a technology perspective.
Have current technologies reached their limits?
In the past, the technologies powering line-system and transponder performance provided networks enough headroom to support incrementally higher degrees of capacity, speed and optical reach. Backward Raman amplification, higher baud-rate transponders and the use of the L-band spectrum have enabled networks to inch closer to the industry’s next significant milestone—efficient terabit networking. But, in their current form, they are not enough.
Raman Amplification
Raman amplifiers allow the ROADM to reduce the gain provided by traditional erbium-doped fiber amplifier (EDFA) amplifiers, to a lower operating point with less noise produced, resulting in a “cleaner” overall signal and longer reach. Many operators deploy a best-of-both-worlds strategy: reduce the noise by lowering the EDFA gain, then recover the gain with Raman amplifiers.
Up to now, Raman amplification has been limited to backward-facing applications in which the Raman amplifier is on the receiving end of the fiber span. In Backward Raman amplification, the pump laser transmits in the direction opposite to the signal propagation.
The holy grail for Raman has been finding a way to successfully deploy the amplification on both ends of the link—Forward and Backward Raman. This combination would allow moving more of the EDFA amplifier gain to the Raman amplifiers, further reducing the EDFA-added noise and further improving the signal’s generalized signal-to-noise ratio (GSNR). The challenge, however, is that Forward Raman also introduces a significant degree of relative intensity noise (RIN) that, in effect, negates the benefits of the technology. Overcoming the added noise has been the key obstacle to unlocking Raman’s full potential—until now. (Spoiler alert).
Transponder technology
Since the introduction of the first coherent optical systems in 2008, transponder technology has continued to advance. The earliest models operated at 10 gigabaud (GBaud) and used quadrature phase-shift keying (QPSK) combined with dual polarization to achieve 4 bits per symbol and a data rate of 40 Gbps. By 2013, 32-Gbaud transponder technology was supporting an effective data rate of about 100 Gbps. When 32 GBaud was paired with 16 QAM modulation and dual polarization, it packed 8 bits per symbol and doubled the data rate to 200Gbps.
“Industry leading companies plan to meet the 1Tb+ challenge by offering 130 – 140Gbaud solution supporting up to 1.2Tbps per wavelength while significantly lowering power per bit.”
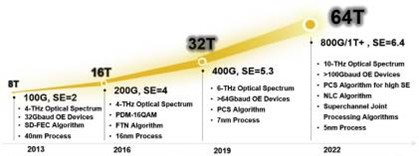
The most recent generation boosts the symbol rate to 90-100 GBaud and operates at up to 800Gbps using 32 QAM. However, with the largest data center customers already contemplating their migration to 1T+ pipes, the question is, how will ICPs and DCOs satisfy the capacity? Industry leading companies plan to meet the 1Tb+ challenge by offering 130 – 140Gbaud solution supporting up to 1.2Tbps per wavelength while significantly lowering power per bit.
Continuous C+L Operation
One more capacity-enabling technology is combining the C and L bands. Terrestrial fiber systems have traditionally operated on the C-band (≈1530nm – 1565nm). But on very high-capacity and constrained routes, this approach was quickly reaching its limits, so the industry started using an adjacent optical spectrum, known as the L band, which runs from approximately 1565nm to 1625nm.
To date, C-band systems have dominated the industry on standard SMF fiber, with L-band being deployed in cases of fiber scarcity or where DSF fiber was installed. Moving forward, the need for additional bandwidth makes using the adjacent L-band, on SMF, which has only minor performance differences from C-band much more appealing.
Traditionally, ICPs and DCOs have tended to rely on their C-band spectrum to serve both single spans (≈80 – 120 km) and regional links (<600 km). As traffic demands build, particularly on the high-capacity links, C-band capacity is quickly maxing out. To handle excess capacity demands, operators have deployed separate “bolt-on” L-band systems and used their control plane to off-load excess traffic to the L band as needed. This bolt-on deployment process is labor-intensive, potentially disruptive and inefficient. Duplicating the add/drop equipment, wavelength selective switch (WSS) and post amps, increases system complexity and management. Additionally, the use of two discrete WSS fabrics complicates management, potentially creating a blocking architecture.
The next-gen optical platform
Fujitsu’s 1FINITY™ 900-Series Optical System represents the next generation of faster, longer, higher-capacity fiber platforms. Designed for terabit networking, it addresses some of the critical obstacles ICPs and DCOs face regarding capacity, speed and reach, as well as various operational challenges. As a result, networks can realize up to 40% more capacity, or up to 40% longer reach, and a significantly lower cost per bit per km.
The 1FINITY 900-Series Optical System is vendor-agnostic. As a result, it can be deployed as either a unified system or a disaggregated solution in which the line system and transponder can be deployed separately.
Solving the Forward Raman challenge
Until recently, overcoming RIN signal degradation has been the major obstacle to successfully implementing Forward Raman. Working with Furukawa Electric Co., Ltd. as key technological partners, Fujitsu has developed a Forward Raman amplifier that minimizes RIN distortion and further reduces the cost per bit per km. This significant achievement now makes it practical and highly beneficial to deploy such amplifiers in optical transport networks to increase optical reachability or fiber capacity.
At the same time, cracking the Forward Raman enigma also addresses the need for greater capacity. Given the same distance, a Forward Raman amplified signal has a higher GSNR. This means a higher-order modulation (more bits per Hz) can be used within the spectral width, and hence enable an overall fiber capacity increase. Based on network and fiber conditions, combined Forward and Backward Raman amplification can increase fiber capacity by up to 40%.
As with all optical network variables, reach and capacity involve tradeoffs in performance. Thus, operators must balance their needs with the understanding that greater reach comes at the expense of more capacity and vice-versa.
135-Gbaud transponder
Another important advancement in the 1FINITY 900-Series Optical System is the introduction of a terabit transponder achieving 1.2T now with seamless upgrade to 1.6T later. The 1.2T transponder features a baud-rate flexible DSP operational up to 135 Gbaud, it enables networks to span any two locations over 6000 km with no regeneration for a 400G wavelength. A higher baud rate enables a higher bit rate – up to 1.2 Tbps on a single wavelength. More capacity translates into fewer wavelengths needed to support client services, hence, less optics and equipment. In other scenarios, an increase in reach can be achieved by lowering the modulation while increasing the baud rate.
Speaking of increasing the baud rate, the 135-GBaud transponder also features programmable baud rate. The ability to dial the baud rate up or down enables operators to completely fill the channel. Spectral margins can also be fine-tuned to account for differences in the channel passband between fiber spans and nodes. This eliminates wasted spectrum while maximizing bit rate and spectral efficiency.
An added advantage of the transponder is the higher density 5nm DSP chip which, when combined with an advanced hybrid liquid-air cooling system, consumes significantly less power than 96-GBaud transponders that use 7 nm chips. As calls for greater sustainability within networks intensify, demonstrable power savings becomes more of a differentiator.
The 150 GHz channel width of the new 135-GBaud technology also simplifies channel planning, enabling networks to allocate spectrum using mutually compatible spectral widths.
Continuous C+L
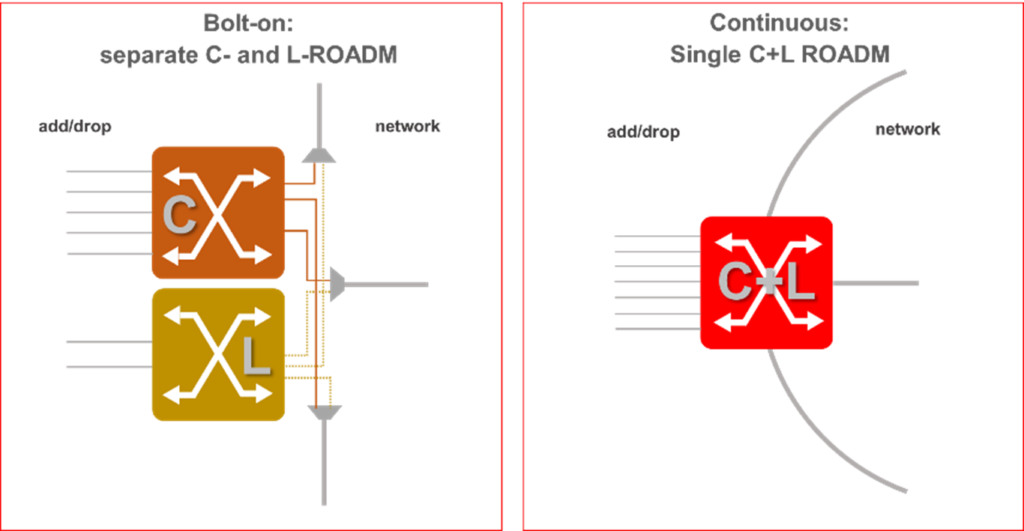
Finally, the Fujitsu 1FINITY 900-Series Optical System enables networks to overcome the operational and management challenges of the traditional bolt-on method for L-band deployment. An advanced WSS combines both C and L bands on a single system that continuously and automatically allocates capacity based on dynamic traffic loads.
The benefit for ICPs and DCOs is the ability to consolidate C- and L-band spectrum equipment into one simplified solution that is easier to deploy and manage and is fully optimized for gain and spectral flatness from Day One. It also results in a higher-efficiency, non-blocking architecture. The technology is expected to be an important step towards simplifying network capacity upgrades requiring the simultaneous use of C band and L band.
It is important to note that, in addition to C+L WDM line systems, 400ZR/ZR+ deployments are well underway. When looking at how to address the capacity issues in their DCIs and cloud applications, ICPs and DCOs can also add pluggable coherent optics into the mix.
Finding the Best Balance of Reach, Capacity, Speed (and Cost)
Fiber transmission is filled with trade-offs, yet it is not an either/or proposition. With the right technology and engineering, operators can achieve a balance in which all variables are improved. This is precisely what the next-gen fiber platforms like the 1FINITY 900-Series Optical System can provide.
For more information on the advanced technologies powering the 1FINITY 900-Series Optical System, we invite you to download Fujitsu’s technical briefs on Forward Raman, 135-GBaud Transponder and Continuous C+L..
Or fill out this form and a Fujitsu representative will contact you: